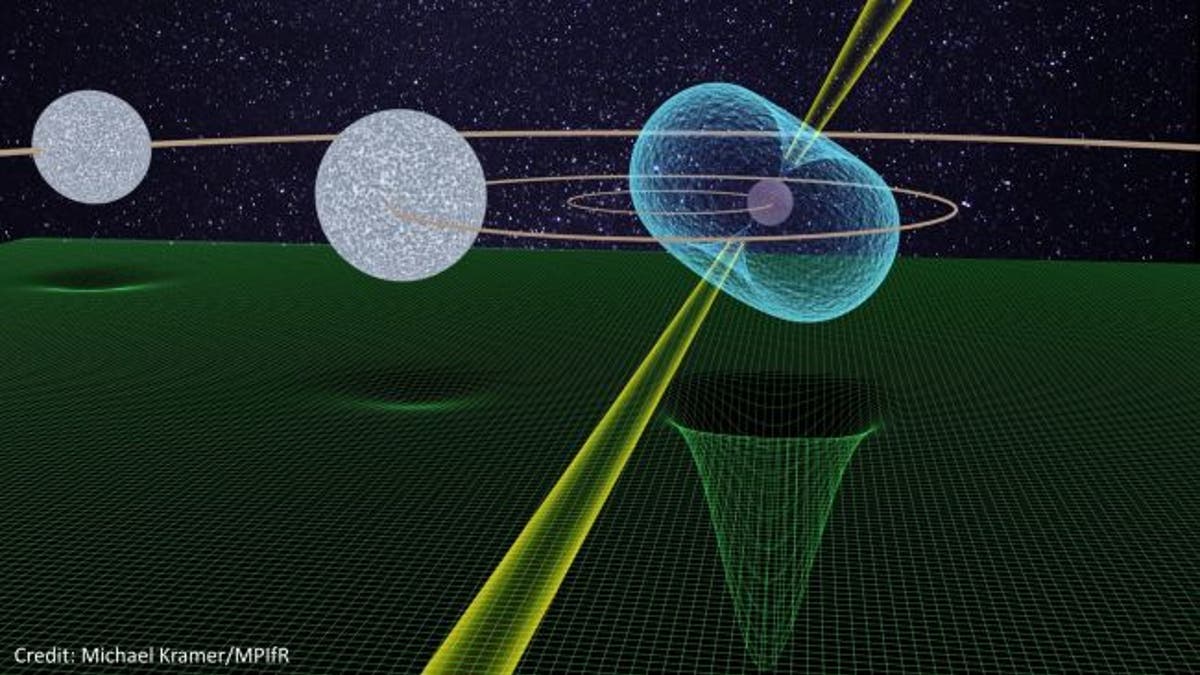
n this illustration, a pulsar (PSR J0337-1715) is shown with two white dwarf companions. The green mesh illustrates the curvature of space-time caused by the different masses. (Size and distances of the three components are not to scale.) (Credit: Michael Kramer/MPIfR)
Once again, physicists have confirmed one of Albert Einstein's core ideas about gravity — this time with the help of a neutron star flashing across space.
The new work makes an old idea even more certain: that heavy and light objects fall at the same rate. Einstein wasn't the first person to realize this; there are contested accounts of Galileo Galilei demonstrating the principle by dropping weights off the Tower of Pisa in the 16th century. And suggestions of the idea appear in the work of the 12th-century philosopher Abu'l-Barakāt al-Baghdādī. This concept eventually made its way into Isaac Newton's model of physics, and then Einstein's theory of general relativity as the gravitational "strong equivalence principle" (SEP). This new experiment demonstrates the truth of the SEP, using a falling neutron star, with more precision than ever.
The SEP has appeared to be true for a long time. You might have seen this video of Apollo astronauts dropping a feather and a hammer in the vacuum of the moon, showing that they fall at the same rate in lunar gravity.
But small tests in the relatively weak gravitational fields of Earth, the moon or the sun don't really put the SEP through its paces, according to Sharon Morsink, an astrophysicist at the University of Alberta in Canada, who wasn't involved in the new study.
"At some level, the majority of physicists believe that Einstein's theory of gravity, called general relativity, is correct. However, that belief is mainly based on observations of phenomena taking place in regions of space with weak gravity, while Einstein's theory of gravity is meant to explain phenomena taking place near really strong gravitational fields," Morsink told Live Science. "Neutron stars and black holes are the objects that have the strongest known gravitational fields, so any test of gravity that involves these objects really test the heart of Einstein's gravity theory."
Neutron stars are the collapsed cores of dead stars. Super dense, but not dense enough to form black holes, they can pack masses greater than that of our sun into whirling spheres just a few miles wide.
The researchers focused on a type of neutron star called a pulsar, which from Earth's perspective seems to flash as it spins. That flashing is a result of a bright spot on the star's surface whirling in and out of view, 366 times per second. This spinning is regular enough to keep time by.
Related: 8 ways you can see Einstein's theory of relativity in real life
This pulsar, known as J0337+1715, is special even among pulsars: It's locked in a tight binary orbit with a white dwarf star. The two stars orbit each other as they circle a third star, also a white dwarf, just like Earth and the moon do as they circle the sun.
(Researchers have already shown that the SEP is true for orbits like this in our solar system: Earth and the moon are affected to exactly the same degree by the sun's gravity, measurements suggest.)
The precise timekeeping of J0337+1715, combined with its relationship to those two gravity fields created by the two white dwarf stars, offers astronomers a unique opportunity to test the principle.
The pulsar is much heavier than the other two stars in the system. But the pulsar still falls toward each of them a little bit as they fall toward the pulsar's larger mass. (The same thing happens with you and Earth. When you jump, you fall back toward the planet very quickly. But the planet falls toward you as well — very slowly, due to your own low gravity, but at the exact same rate as a feather or a hammer would if you ignore air resistance.) And because J0337+1715 is such a precise timekeeper, astronomers on Earth can track how the gravitational fields of the two stars affect the pulsar's period.
To do so, the astronomers carefully timed the arrival of light from J0337+1715 using large radio telescopes, in particular the Nançay Radio Observatory in France. As the star moved around each of its neighbors — one in a quick little orbit and one in a longer, slower orbit — the pulsar got closer and farther from Earth. As the neutron star moved farther away from Earth, the light from its pulses had to travel longer distances to reach the telescope. So, to a tiny degree, the gaps between the pulses seemed to get longer.
As the pulsar swung back toward Earth, the gaps between the pulses got shorter. That allowed physicists to build a robust model of the neutron star's movement through space, explaining precisely how it interacted with the gravity fields of its neighbors. Their work built on a technique used in an earlier paper, published in the journal Nature in 2018, to study the same system.
The new paper, published online June 10 in the journal Astronomy and Astrophysics, showed that the objects in this system behaved as Einstein's theory predicts — or at least didn't differ from Einstein's predictions by more than 1.8 parts per million. That's the absolute limit of the precision of their telescope data analysis. They reported 95% confidence in their findings.
Morsink, who uses X-ray data to study the mass, widths, and surface patterns of neutron stars, said that this confirmation isn't surprising, but it is important for her research.
"In that work, we have to assume that Einstein's theory of gravity is correct, since the data analysis is already very complex," Morsink told Live Science in an in an email. "So tests of Einstein's gravity using neutron stars really make me feel better about our assumption that Einstein's theory describes the gravity of a neutron star correctly!"
Without understanding the SEP, Einstein would never have been able to develop his ideas of relativity. In an insight he described as "the most fortunate thought in my life," he recognized that objects in free fall don't feel the gravitational fields tugging on them.
(This is why astronauts in orbit around the Earth float. In constant free fall, they don't experience the gravitational field that holds them in orbit. Without windows, they wouldn't know Earth was there at all.)
Most of Einstein's key insights about the universe begin with the universality of free fall. So, in this way, the cornerstone of general relativity has been made that much stronger.
- 15 amazing images of stars
- 11 fascinating facts about the Milky Way
- The 18 biggest unsolved mysteries in physics
Originally published on Live Science.