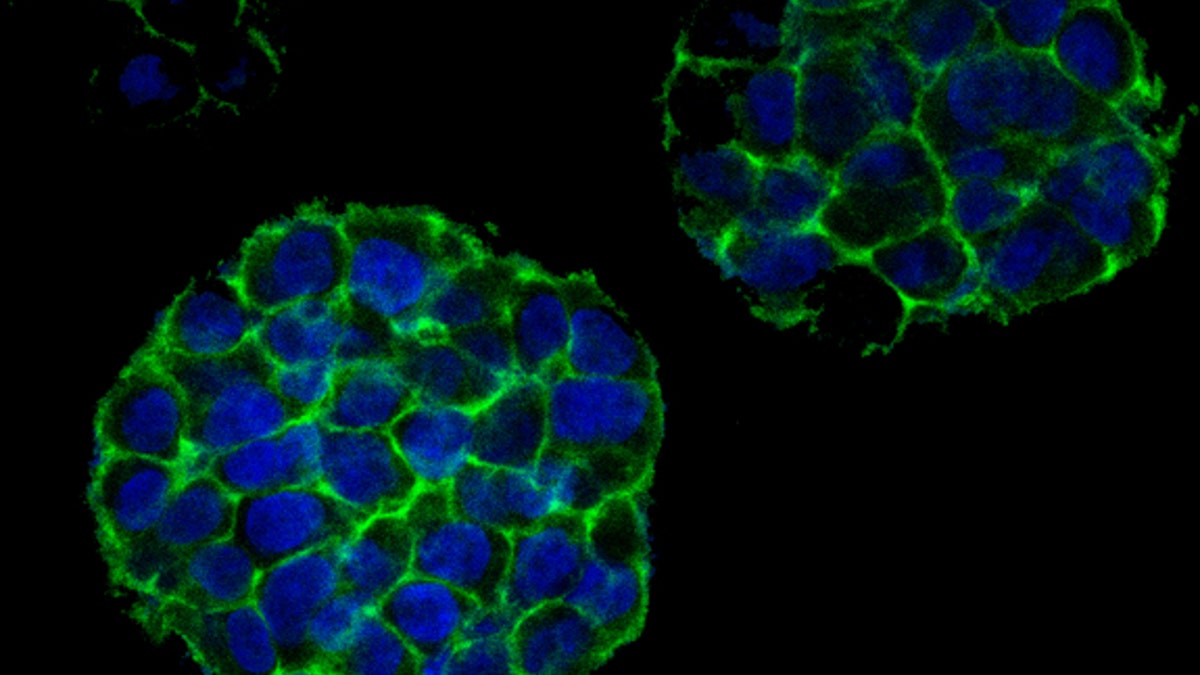
3D printed HeLa cells in tumor-like configurations (Zhao et al. Biofabrication, 2014)
With everything from violins to rifles being made on 3d printers, it seems the devices have taken the notion of DIY to a whole other level.
Now, 3D printing is allowing scientists to gain insights into some of the tiniest constituents of the universe: biological molecules.
Although researchers have used computer models to visualize the origami-like process of protein folding for years, "the experience itself is very different between looking at something on a flat screen, and actually holding an object and manipulating an object in your hands," said Arthur Olson, a molecular biologist at the Molecular Graphics Laboratory at the Scripps Research Institute in La Jolla, California. [10 Weirdest Things Created By 3D Printing]
This could one day help researchers design new drugs that more precisely target spots on virus molecules or even manufacture artificial protein sensors.
3d printing
3D printing has become incredibly useful in several areas of medical science: 3d-printed hearts, livers and skulls are already in use to help doctors plan surgeries, and may even save lives. The technology has also been used to print synthetic ears, blood vessels and sheets of cardiac muscle that really beat.
But the printing technique is also helping scientists in the basic sciences.
Olson is using the 3D-printed models to understand how HIV, the virus that causes AIDS, functions. He is sharing his models with other researchers through the National Institutes of Health's 3d print exchange, a program that allows scientists to share instructions for printing molecules, organs and other objects.
Proteins often contain thousands of atoms. That can make it difficult to see how proteins fold up, or how the myriad forces between individual molecules interact, Olson said.
But 2D computer visualizations have limitations that make them hard to interpret. For instance, when researchers try to move molecules around in computer simulations, they often go right through each other, which wouldn't happen in the physical world, Olson said.
With a 3D model, there's no way for two solid molecules to go right through each other, he said.
The printing method also reveals new insights when two molecules interact. For instance, many proteins have long, curvy tunnels within them, through which molecules pass. Determining a tunnel's length and width can be very tricky on the computer screen because there's no way to see all the way through from any one view. But measuring its length is exceedingly easy in 3d-printed models, Olson said.
"All you have to do is take string, push it through the tunnel, mark the ends, stretch it out, and you know how long the tunnel is," Olson told Live Science.
Future molecules
3D printing could also be used to design completely artificial molecules. Proteins are very good at detecting molecules, such as small concentrations of a poison or explosives in a subway, but proteins don't do well in hot, cold, dry or other extreme conditions, said Ron Zuckerman, a nanobioscientist at the Molecular Foundry at Berkeley Lab in California.
So Zuckerman is developing synthetic molecules called "peptoids." These molecules would have the sensitivity of proteins, but could be made of stronger and more rugged synthetic amino acids.
His team began using 3D printing because it provides researchers a more intuitive way to understand how flexible proteins are, which makes it easier to understand how they fold. The attractive and repulsive forces between molecules can be modeled with tiny magnets on the models, and materials with different flexibility can mimic the bendiness of different protein structures.
Zuckerman is currently using printed models of real proteins that he calls "peppytides" for educational purposes, showing how structures that are common to many proteins, such as the telephone-cordlike structure called an alpha-helix, emerge.
When students start with a 3D model, "I can give you this floppy thing like a necklace that's just wiggling -around and you can actually fold it," Zuckerman told Live Science. "All of a sudden the helical folds start to become stable because all the magnets line up."
- 7 Cool Uses of 3D Printing in Medicine
- Image Gallery: Stunning Peek Inside Molecules
- Photos: 3D Printed Hearts
Copyright 2014 LiveScience, a TechMediaNetwork company. All rights reserved. This material may not be published, broadcast, rewritten or redistributed.