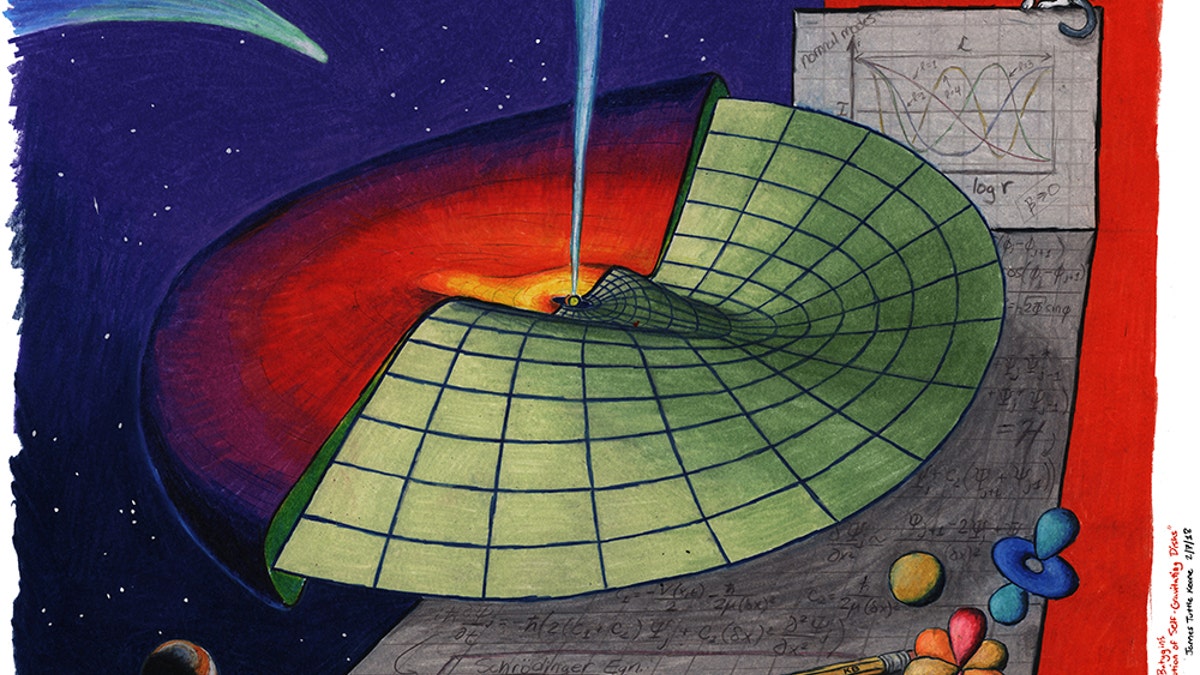
New research ties the warping of large-scale disks of material in the universe with the Schrodinger equation, which describes the quantum mechanical behavior of atomic and subatomic objects. (James Tuttle Keane/California Institute of Technology)
Enormous disks of stars or debris can operate under the same rules as subatomic particles, changing based on the Schrodinger equation, which physicists use to model quantum-mechanical systems.
Viewing space structures with that equation can lend new insights into how galaxies evolve, as well as reveal clues about the mechanics of the early solar system and the action of rings circling distant planets, a new study reports.
California Institute of Technology researcher Konstantin Batygin, author of the new study, hadn't expected to find that particular equation when studying those astrophysical disks. "At the time, I was completely floored," Batygin told Space.com. "I was expecting the regular wave equation to appear, something like the wave of a string or something like that. And instead, I get this equation, which is really the cornerstone of quantum mechanics." [Planet-Building 'Flying Saucer' Disk Is Surprisingly Cool (Video)]
Using the Schrodinger equation, physicists can interpret the interactions of systems on atomic and subatomic scales in terms of waves as well as particles — a key concept in quantum mechanics that describes those systems' sometimes unintuitive behavior. It turns out, the warping of astrophysical disks can act like particles, too.
More From Space.com
"In retrospect, when I look at the problem now, I'm surprised at how I didn't just guess that that's what it was going to be," said Batygin, who's perhaps best known (to laypeople, anyway) for co-authoring a 2016 study with fellow Caltech researcher Mike Brown that found evidence for a possible undiscovered "Planet Nine" in the dark depths of our outer solar system.
Blast from the past
Batygin came across the connection when teaching a class. He was trying to explain how waves travel through the broad disks that are a staple of space architecture — for instance, such disks are built of stars around supermassive black holes at a galaxy's center, and made of dust and debris in a newborn star system. The disks bend and warp in a complex way that current modeling can't handle on all timescales. Scientists can calculate their actions over very short time spans, like what happens over a few orbits, as well as how they will disperse over an entire lifetime, but not how and why they will change on the order of hundreds of thousands of years.
"Things might happen, and you don't really know why — it's a complicated system, so you just see stuff unfold, see some kind of dynamical evolution unfold," Batygin said. "Unless you have this monstrously complicated physical intuition, you just don't understand what's going on in your simulation."
To follow a disk's development, Batygin borrowed a trick from the 1770s: calculating the way mathematicians Joseph-Louis Lagrange and Pierre-Simon Laplace modeled the solar system as a series of giant loops following the planets' orbits. While the model wasn't helpful on short timescales of a few circuits around the sun, it could accurately depict the orbits' interactions with one another over time.
Instead of modeling individual planets' orbits, Batygin used a series of thinner and thinner rings to represent different pieces of the astrophysical disk, like layers of an onion, each tied to the mass of the orbiting bodies within that region.The rings' gravitational interactions with one another could model how the disk would warp and change.
And when the system got too complicated to calculate by hand or on the computer as he added more rings, he used a mathematical shortcut to convert to describing an infinite number of infinitely thin rings.
"This is just a broadly known mathematical result which is used in physics left and right," Batygin said. But yet, somehow, nobody had taken the leap to model an astrophysical disk that way.
"What is truly remarkable to me is that nobody has blurred [the rings] into a continuum ever before," he said. "It seems so obvious in retrospect, and I don't know why I didn't think of it sooner."
A remarkable connection
When Batygin went through those calculations, he found the emerging equation surprisingly familiar.
"Of course, the two are related, right? In quantum mechanics, you treat particles as waves," he said. "In retrospect, it's kind of almost intuitive that you should get something like the Schrodinger equation, but at the time, I really was genuinely surprised." The equation has popped up unexpectedly before, he added — in descriptions of ocean waves, for instance, as well as the way light moves through certain nonlinear media.
"What my research demonstrates is that the long-term behavior of astrophysical disks, the way in which they bend and warp, joins this group of classical contexts that can be understood in an essentially quantum framework," Batygin said.
The new results raise an interesting analogy between the two situations: The way waves travel through astrophysical disks, bouncing off the inner and outer edges, is equivalent to how a single quantum particle bounces back and forth between two walls, he said.
Finding this equivalence has one interesting consequence: Batygin was able to borrow some of the work done by researchers who have already studied and worked through this quantum situation extensively, and then interpret the equation in this new context to understand how disks respond to external pulls and perturbations.
"Physicists have a lot of experience with the Schrodinger equation; it's coming up on 100 years old now," Greg Laughlin, an astrophysicist at Yale University who was not involved with the study, told Space.com. "And a lot of very deep thought has gone into understanding its ramifications. And so that whole edifice now can be applied to the evolution of disks."
"And for someone like me — who has admittedly a better sense, although imperfect, of what protostellar disks do — this also gives the opportunity to go the other way and maybe get some deeper insight into quantum systems by using the disk analogy," he added. "I do think that it's going to spark a lot of attention, and interest, probably consternation. And ultimately I think it's going to be a really interesting development."
A framework of understanding
Batygin looks forward to applying the equation to understanding many different facets of astrophysical disks.
"What I have presented in this paper is a framework," Batygin said. "I have attacked one particular problem with it, which is the problem of disk rigidity — the extent to which the disk can remain gravitationally rigid under external perturbations. There's a broad range of additional applications that I'm looking into at the moment."
One example is the evolution of the disk of debris that eventually formed our solar system, Batygin said. Another is the dynamics of rings around extrasolar planets. And a third is the disk of stars surrounding the black hole at the center of the Milky Way, which itself is highly bent.
Laughlin noted that the work should be particularly helpful in improving researchers' understanding of newborn star systems because they're harder to observe from afar, and researchers currently can't simulate their development from start to end.
"The mathematical framework that Konstantin has put together is a good example of something that might really help us understand how objects that are hundreds of thousands of orbits old, like a planet-forming disk, behave," he said.
According to Fred Adams, an astrophysicist at the University of Michigan who was not involved in the study, this new work is most useful for systems in which large-scale gravity effects cancel out. For systems with more complicated gravitational influences, like galaxies with very distinct spiral arms, some other modeling strategy will be needed. But for this class of problem, it's an interesting variation on approximating waves in astrophysical disks, he said.
"Research in any field, including circumstellar disks, always benefits from the development and use of new tools," Adams said. "This paper represents the development of a new analytic tool, or a new twist on older tools, depending on how you look at it. Either way, it's another piece of the larger puzzle."
The framework will let researchers understand the structures astronomers see in the night sky in a new way: While these disks are changing on far longer timescales than humans can observe, the equation can be applied to figure out how a system got to the point we see today and how it might change in the future, Batygin said. And it's all based on math that usually describes incredibly quick, fleeting interactions.
"There's this intriguing reciprocity between the mathematics that govern the behavior of the subatomic world and the mathematics that governs the behavior [and] long-term evolution of these astronomical things that unfold on much, much longer timescales," he added. "That, I think, is a remarkable and intriguing consequence."
The new work was detailed today (March 5) in the journal Monthly Notices of the Royal Astronomical Society.
Original article on Space.com.